Real-Life Tony Stark: Iron Man’s Arc Reactor
- joann yu
- Jul 3, 2023
- 3 min read
We all know Iron Man’s (the best Avenger) iconic suit: brilliant gold and a little hot rod red. What is almost just as iconic is the glowing, mini arc reactor resting over Robert Downey Jr’s chest. No matter how sophisticated Tony Stark’s maneuvers seemed in his makeshift “lab” as he was held hostage in Iron Man I (the best Marvel film), the ability to create such a miniature, fusion-driven device is truly science fiction (emphasis on the fiction). Even the larger arc reactors that ran continuously in later films are not currently operational in the real world.
Fusion energy is an energy source based on a controlled thermonuclear fusion reaction: the process occurs when two nuclei (plural of nucleus) of distinct atoms collide and combine to form a new nucleus. In terms of natural systems, fusion reactions are what occur every second in the sun. The proton-proton fusion of two hydrogen atoms to make helium is the main process that powers stars; it also allows our sun to radiate throughout our solar system.
When nuclei are crushed together, they release an immense amount of energy. Indeed, fusion releases energy because the mass of the final nucleus is less than the mass of the previous nuclei–the mass deficit is converted to energy through Einstein’s equation, E=mc2. However, because the nuclei are positively charged, they repel each other, so it takes tremendous pressures and temperatures to overcome that electrostatic barrier and get them to merge.
Creating conditions for fusion on Earth involves generating and sustaining plasma. Plasmas are gasses that are so hot that electrons move fast enough to free them from the electromagnetic pull of their atom’s nucleus. When sufficiently high temperatures are reached and ions overcome the repulsive electrostatic forces and fuse together, they form a plasma. You may have heard tons of news about fusion during December of 2022, when for the first time, researchers at Lawrence Livermore National Laboratory in California were able to achieve “net energy,” meaning that they extracted more energy from a fusion reaction than the energy used to trigger it.
Currently, fusion reactors generally use heavy hydrogen isotopes such as deuterium and tritium which react more easily than protium (the most common hydrogen isotope), as they require less extreme conditions.
Earth abounds with deuterium: the isotope constitutes 0.016 percent of natural hydrogen, so the seas are flooded in it–we have enough supplies to last millions of years. But tritium is a paucity, and its radioactive decay half-life is just 12 years, so it's constantly disappearing. In principle, it can be “created” from fusion reactions because the fusion neutrons will react with lithium (extracted from the crust of the earth) to make it. Most reactors incorporate this “breeding” process by designing the reactor chamber with a lithium blanket. However, this technology is untested at large scales in the long term, and no one knows how well tritium production will work in practice.
The energy released in fusion can theoretically be self-sustaining. But how do you bottle plasma at a temperature of 100 million kelvins, which is several times hotter than the core of the sun?
The answer proposed is magnetic confinement: holding the electrically charged plasma in a structure formed by powerful magnetic fields so it never touches the walls of the fusion chamber. The most popular design for fusion reactors is the tokamak, a doughnut-shaped container, which was proposed in the 1950s by Soviet scientists. However, even with the invention of magnetic-confinement reactors, the necessary combination of intense temperature and pressure for fusion reactions has proven to be difficult to sustain reasonably and economically.

Credits: Scientific American
The magnetic-confinement design of fusion reactors faces a significant obstacle: the need for materials that can withstand the constant bombardment they'll receive from the fusing plasma. Deuterium-tritium fusion in particular creates severe fluxes of high-energy neutrons, which in turn collide with the atoms in the metal walls. Such extreme conditions would melt even extremely heat-resistant metals, such as tungsten. Indeed, the aftermath of such an onslaught of neutron pelting isn't well documented, as fusion has never been sustained for the long periods that would occur in a working reactor.
See Part II...
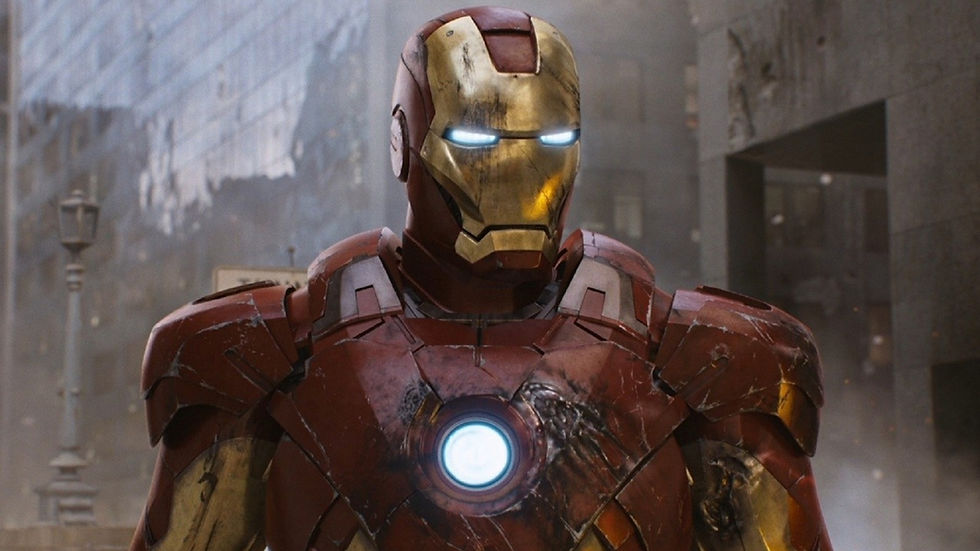
Comments